Research
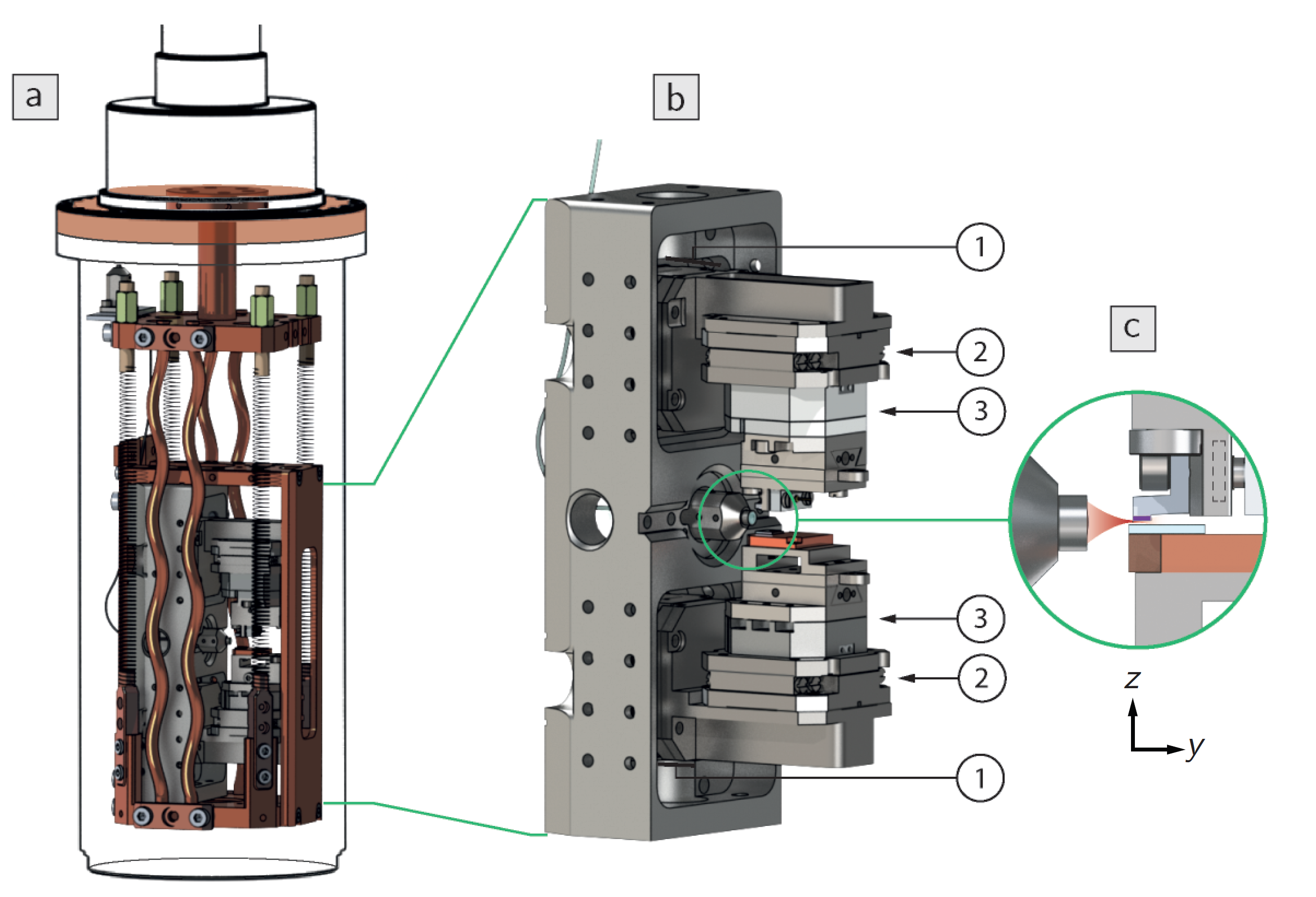
Our research group focuses on nanometer-scale mechanics, magnetism, and imaging. We explore the ultimate thermal and quantum limits of mechanical sensors, study how magnetic materials behave on the nanometer-scale, and the use sensitive imaging tools to investigate magnetization patterns, spin configurations, and current distributions.
Our group has established itself as a leader in sensitive force microscopy – in particular as applied to nuclear magnetic resonance (NMR) – in the mechanics of nanowires (NWs), in the magnetism of ferromagnetic nanotubes (FNTs), in hybrid mechanical systems, and in microscopy with nanometer-scale superconducting quantum interference devices (SQUIDs). We have been at the forefront in developing measurements techniques such as NW-based atomic force microscopy (AFM) and magnetic force microscopy (MFM), sensitive torque magnetometry, and scanning quantum dot (QD) microscopy. We are also among the few groups in the world with the capability to fabricate and use SQUID-on-tip sensors for nanometer-scale magnetic and thermal imaging. More recently we have been developing scanning SQUID microscopy with SQUID-on-lever sensors, combining magnetic, thermal, and topographic contrast.
The imaging techniques employed by our group do not only resolve exquisitely weak patterns, including those generated by single charges, spins, and small amounts of flowing current, but they also probe systems at nanometer length-scales. Such localized measurements, unlike global magnetization or transport measurements, allow us to overcome ensemble or spatial inhomogeneity in systems ranging from magnetic nanotubes, to superconducting thin films, to strongly correlated states in van der Waals heterostructures. This capability is the key to unravelling the microscopic mechanisms behind a wealth of new and poorly understood condensed matter phenomena.
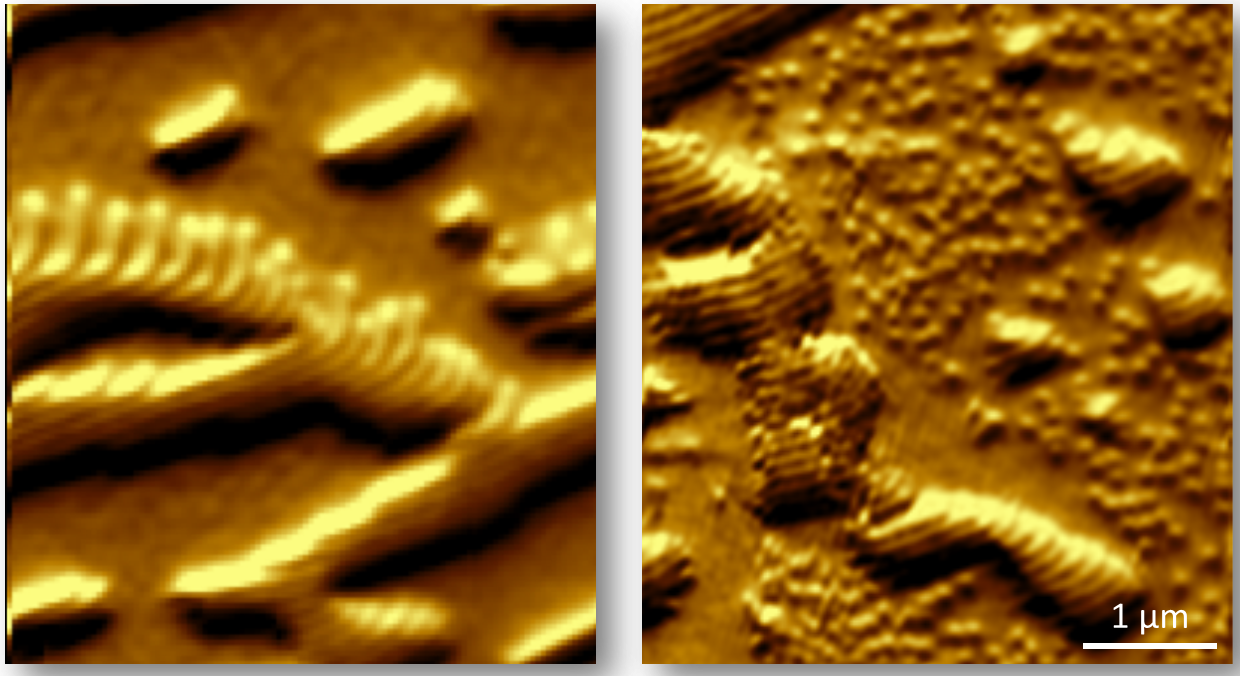
Key Results
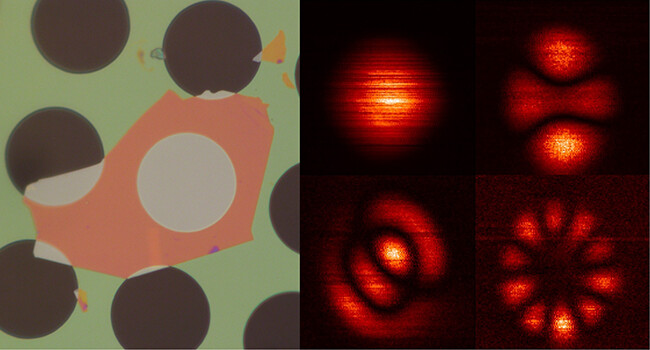
Nanomechanics, optomechanics, and hybrid systems
We study the mechanical motion of a number of nanomechanical resonators including NWs, carbon nanotubes, and 2D membranes. Early efforts centered on the mechanical nonlinearities inherent in NW flexural motion as well as the coupling between a NW’s mechanical motion and optically active QDs embedded inside. Current efforts are aimed at studying the optomechanics of hBN membranes and carbon nanotubes.
References
- Coupling trapped ions to a nanomechanical oscillator
M. Weegen, M. Poggio, and S. Willitsch
arXiv:2312.00576. (pdf)
- Mechanical mode imaging of a high-Q hybrid hBN/Si3N4 resonator
D. Jaeger, F. Fogliano, T. Ruelle, A. Lafranca, F. R. Braakman, and M. Poggio
Nano Lett. 23, 2016 (2023). (pdf) (pdf)
- A tunable fiber Fabry-Perot cavity for hybrid optomechanics stabilized at 4 K
T. Ruelle, D. Jaeger, F. Fogliano, F. R. Braakman, and M. Poggio
Rev. Sci. Instrum. 93, 095003 (2022). (pdf)
- Nanowire magnetic force sensors fabricated by focused-electron-beam-induced deposition
H. Mattiat, N. Rossi, B. Gross, J. Pablo-Navarro, C. Magén, R. Badea, J. Berezovsky, J. M. De Teresa, and M. Poggio
Phys. Rev. Appl. 13, 044043 (2020). (pdf)
- Force sensing with nanowire cantilevers
F. R. Braakman and M. Poggio
Nanotechnology 30, 332001 (2019). (pdf)
- Optimized single-shot laser ablation of concave mirror templates on optical fibers
T. Ruelle, M. Poggio, and F. R. Braakman
Appl. Opt. 58, 3784 (2019). (pdf)
- Magnetic force sensing using a self-assembled nanowire
N. Rossi, B. Gross, F. Dirnberger, D. Bougeard, and M. Poggio
Nano Lett. 19, 930 (2019). (pdf) (pdf)
- Classical and quantum dynamics of a trapped ion coupled to a charged nanowire
P. Fountas, M. Poggio, and S. Willitsch
New J. Phys. 21, 013030 (2019). (pdf)
- Coherent two-mode dynamics of a nanowire force sensor
F. R. Braakman, N. Rossi, G. Tütüncüoglu, A. Fontcuberta i Morral, and M. Poggio
Phys. Rev. Appl. 9, 054045 (2018). (pdf)
- Resonant driving of a single photon emitter embedded in a mechanical oscillator
M. Munsch, A. V. Kuhlmann, D. Cadeddu, J.-M. Gérard, J. Claudon, M. Poggio, and R. J. Warburton
Nat. Commun. 8, 76 (2017). (pdf)
- Vectorial scanning force microscopy using a nanowire resonator
N. Rossi, F. R. Braakman, D. Cadeddu, D. Vasyukov, G. Tütüncüoglu, A. Fontcuberta i Morral, and M. Poggio
Nat. Nanotechnol. 12, 150 (2017). (pdf) (pdf)
- Time-resolved nonlinear coupling between orthogonal flexural modes of a pristine GaAs nanowire
D. Cadeddu, F. R. Braakman, G. Tütüncüoglu, F. Matteini, D. Rüffer, A. Fontcuberta i Morral, and M. Poggio
Nano Lett., 16, 926 (2016). (pdf) (pdf)
- Permanent reduction of dissipation in nanomechanical Si resonators by chemical surface protection
Y. Tao, P. Navaretti, R. Hauert, U. Grob, M. Poggio, and C. L. Degen
Nanotechnology 26, 465501 (2015). (pdf) (pdf)
- Nonlinear motion and mechanical mixing in as-grown GaAs nanowires
F. R. Braakman, D. Cadeddu, G. Tütüncüoglu, F. Matteini, D. Rüffer, A. Fontcuberta i Morral, and M. Poggio
Appl. Phys. Lett. 105, 173111 (2014). (pdf)
- Quantum dot opto-mechanics in a fully self-assembled nanowire
M. Montinaro, G. Wüst, M. Munsch, Y. Fontana, E. Russo-Averchi, M. Heiss, A. Fontcuberta i Morral, R. J. Warburton, and M. Poggio
Nano Lett. 14, 4454 (2014). (pdf) (pdf)
- Hybrid Mechanical Systems
P. Treutlein, C. Genes, K. Hammerer, M. Poggio, and P. Rabl
Cavity Optomechanics, M. Aspelmeyer, T. Kippenberg, F. Marquardt, Eds., Springer (2014), pp. 327-351. (pdf)
- Feedback cooling of cantilever motion using a quantum point contact transducer
M. Montinaro, A. Mehlin, H. S. Solanki, P. Peddibhotla, S. Mack, D. D. Awschalom, and M. Poggio
Appl. Phys. Lett. 101, 133104 (2012). (pdf)
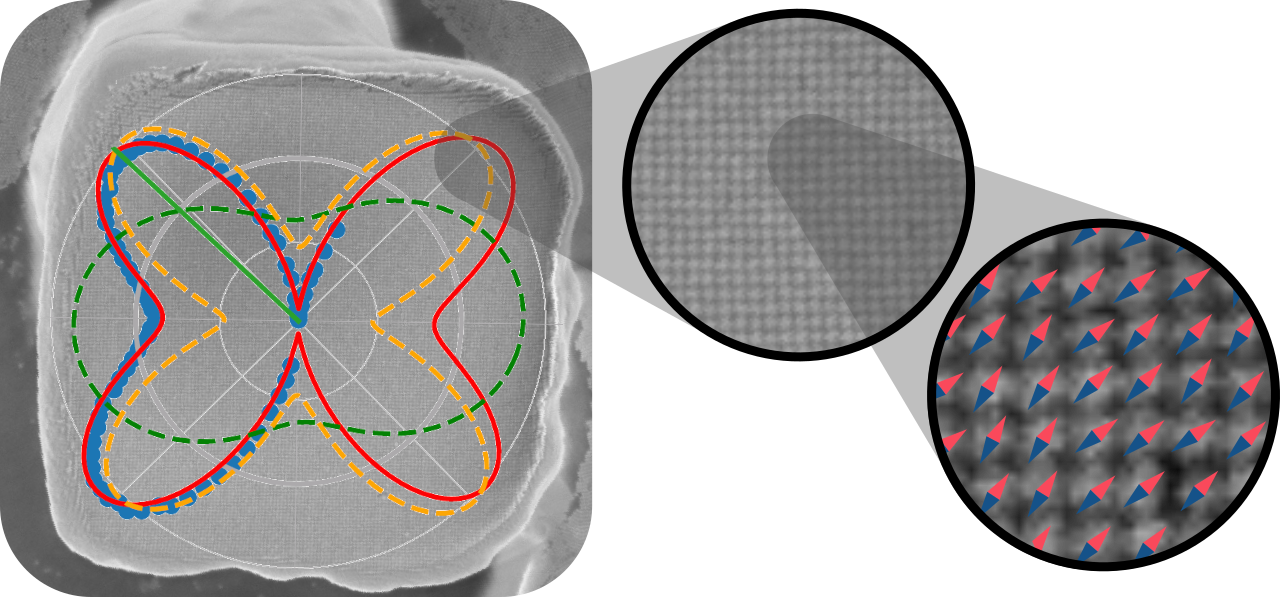
Ultrasensitive torque magnetometry
We develop sensitive torque magnetometry to study particularly weak magnetic effects or magnetism in small samples. We combine such measurements with micro-magnetic simulations to reveal magnetization configurations, reversal behaviors, and magnetic phases. We have measured the hysteresis of individual nanomagnets, identified magnetic phase transitions in helimagnets, and found evidence for previously unknown magnetic states. The power of this technique lies in its ability to determine magnetic anisotropy and in its high sensitivity to tiny magnetizations. These properties, together with the sensitivity of magnetic torque to phase transitions, make it ideal for the measurement of 2D magnets.
References
- Magnetic properties of an individual Magnetospirillum gryphiswaldense cell
M. M. Claus, M. Wyss, D. Schüler, M. Poggio, and B. Gross
arXiv:2403.11801. (pdf)
- Magnetic hysteresis of individual Janus particles with hemispherical exchange biased caps
S. Philipp, B. Gross, M. Reginka, M. Merkel, M. M. Claus, M. Sulliger, A. Ehresmann, and M. Poggio
Appl. Phys. Lett. 119, 222406 (2021). (pdf) (pdf)
- Magnetic anisotropy of individual maghemite mesocrystals
B. Gross, S. Philipp, E. Josten, J. Leliaert, E. Wetterskog, L. Bergström, and M. Poggio
Phys. Rev. B 103, 014402 (2021). (pdf)
- Stability of Néel-type skyrmion lattice against oblique magnetic fields in GaV4S8 and GaV4Se8
B. Gross, S. Philipp, K. Geirhos, A. Mehlin, S. Bordács, V. Tsurkan, A. Leonov, I. Kézsmárki, and M. Poggio
Phys. Rev. B 102, 104407 (2020). (pdf)
- Macroscopic manifestation of domain-wall magnetism and magnetoelectric effect in a Néel-type skyrmion host
K. Geirhos, B. Gross, B. G. Szigeti, A. Mehlin, S. Philipp, J. S. White, R. Cubitt, S. Widmann, S. Ghara, P. Lunkenheimer, V. Tsurkan, A. O. Leonov, S. Bordács, M. Poggio, and I. Kézsmárki
npj Quantum Mater. 5, 44 (2020). (pdf) (pdf)
- Determining magnetization configurations and reversal of individual magnetic nanotubes
M. Poggio
Magnetic Nano- and Microwires (Second Edition), M. Vázquez, Ed., Elsevier (2020), pp. 491-517. (pdf)
- Observation of end-vortex nucleation in individual ferromagnetic nanotubes
A. Mehlin, B. Gross, M. Wyss, T. Schefer, G. Tütüncüoglu, F. Heimbach, A. Fontcuberta i Morral, D. Grundler, and M. Poggio
Phys. Rev. B 97, 134422 (2018). (pdf)
- Dynamic cantilever magnetometry of individual CoFeB nanotubes
B. Gross, D. P. Weber, D. Rüffer, A. Buchter, F. Heimbach, A. Fontcuberta i Morral, D. Grundler, and M. Poggio
Phys. Rev. B 93, 064409 (2016). (pdf)
- Magnetization reversal of an individual exchange-biased permalloy nanotube
A. Buchter, R. Wölbing, M. Wyss, O. F. Kieler, T. Weimann, J. Kohlmann, A. B. Zorin, D. Rüffer, F. Matteini, G. Tütüncüoglu, F. Heimbach, A. Kleibert, A. Fontcuberta i Morral, D. Grundler, R. Kleiner, D. Koelle, and M. Poggio
Phys. Rev. B 92, 214432 (2015). (pdf) (pdf)
- Stabilized skyrmion phase in MnSi nanowires detected by dynamic cantilever magnetometry
A. Mehlin, F. Xue (薛飞), D. Liang, H. Du, M. J. Stolt, S. Jin, M. Tian, and M. Poggio
Nano Lett. 15, 4839 (2015). (pdf) (pdf)
- Vortex lattice melting of a NbSe2 single grain probed by ultrasensitive cantilever magnetometry
L. Bossoni, P. Carretta, and M. Poggio
Appl. Phys. Lett. 104, 182601 (2014). (pdf)
- Nanoscale multifunctional sensor formed by a Ni nanotube and a scanning Nb nanoSQUID
J. Nagel, A. Buchter, F. Xue (薛飞), O. F. Kieler, T. Weimann, J. Kohlmann, A. B. Zorin, D. Rüffer, E. Russo-Averchi, R. Huber, P. Berberich, A. Fontcuberta i Morral, D. Grundler, R. Kleiner, D. Koelle, M. Poggio, and M. Kemmler
Phys. Rev. B 88, 064425 (2013). (pdf)
- Reversal mechanism of an individual Ni nanotube simultaneously studied by torque and SQUID magnetometry
A. Buchter, J. Nagel, D. Rüffer, F. Xue (薛飞), D. P. Weber, O. F. Kieler, T. Weimann, J. Kohlmann, A. B. Zorin, E. Russo-Averchi, R. Huber, P. Berberich, A. Fontcuberta i Morral, M. Kemmler, R. Kleiner, D. Koelle, D. Grundler, and M. Poggio
Phys. Rev. Lett. 111, 067202 (2013). (pdf) (pdf)
- Cantilever magnetometry of individual Ni nanotubes
D. P. Weber, D. Rüffer, A. Buchter, F. Xue (薛飞), E. Russo-Averchi, R. Huber, P. Berberich, J. Arbiol, A. Fontcuberta i Morral, D. Grundler, and M. Poggio
Nano Lett. 12, 6139 (2012). (pdf) (pdf)
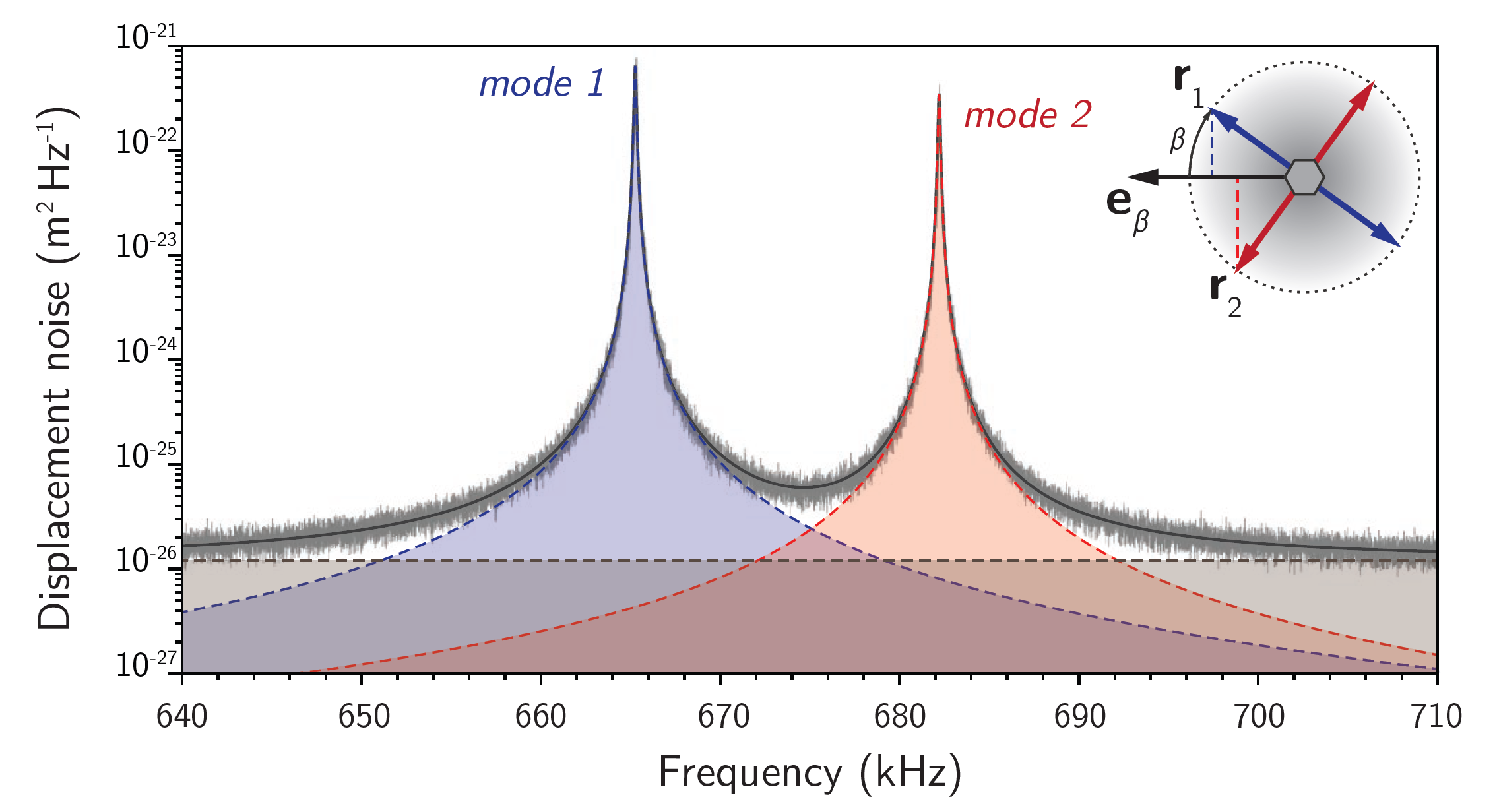
Nanowires as force sensors and scanning probes
We have pioneered the use of NWs as mechanical transducers and scanning sensors. These tiny resonators can have exceptional properties, including small motional mass, high resonant frequency, and low dissipation. We have learned how to actuate and detect their motion and use them as scanning probes, such than an individual NW acts like a tiny mechanical compass that measures both the direction and size of the surrounding forces. Using these devices, we have demonstrated new types of ultrasensitive AFM and MFM. Recently, we have put such sensors to work investigating subtle magnetization distributions in 2D magnets.
References
- Mapping the phase-separated state in a 2D magnet
H. Mattiat, L. Schneider, P. Reiser, M. Poggio, P. Sahafi, A. Jordan, R. Budakian, D. V. Averyanov, I. S. Sokolov, A. N. Taldenkov, O. E. Parfenov, O. A. Kondratev, A. M. Tokmachev, and V. G. Storchak
Nanoscale, DOI: 10.1039/D3NR06550B (2024). (pdf) (pdf)
- Coupling trapped ions to a nanomechanical oscillator
M. Weegen, M. Poggio, and S. Willitsch
arXiv:2312.00576. (pdf)
- Nanoscale magnetic field imaging for 2D materials
E. Marchiori, L. Ceccarelli, N. Rossi, L. Lorenzelli, C. L. Degen, and M. Poggio
Nat. Rev. Phys. 4, 49 (2022). (pdf)
- Nanowire magnetic force sensors fabricated by focused-electron-beam-induced deposition
H. Mattiat, N. Rossi, B. Gross, J. Pablo-Navarro, C. Magén, R. Badea, J. Berezovsky, J. M. De Teresa, and M. Poggio
Phys. Rev. Appl. 13, 044043 (2020). (pdf)
- Force sensing with nanowire cantilevers
F. R. Braakman and M. Poggio
Nanotechnology 30, 332001 (2019). (pdf)
- Magnetic force sensing using a self-assembled nanowire
N. Rossi, B. Gross, F. Dirnberger, D. Bougeard, and M. Poggio
Nano Lett. 19, 930 (2019). (pdf) (pdf)
- Classical and quantum dynamics of a trapped ion coupled to a charged nanowire
P. Fountas, M. Poggio, and S. Willitsch
New J. Phys. 21, 013030 (2019). (pdf)
- Coherent two-mode dynamics of a nanowire force sensor
F. R. Braakman, N. Rossi, G. Tütüncüoglu, A. Fontcuberta i Morral, and M. Poggio
Phys. Rev. Appl. 9, 054045 (2018). (pdf)
- Vectorial scanning force microscopy using a nanowire resonator
N. Rossi, F. R. Braakman, D. Cadeddu, D. Vasyukov, G. Tütüncüoglu, A. Fontcuberta i Morral, and M. Poggio
Nat. Nanotechnol. 12, 150 (2017). (pdf) (pdf)
- Time-resolved nonlinear coupling between orthogonal flexural modes of a pristine GaAs nanowire
D. Cadeddu, F. R. Braakman, G. Tütüncüoglu, F. Matteini, D. Rüffer, A. Fontcuberta i Morral, and M. Poggio
Nano Lett., 16, 926 (2016). (pdf) (pdf)
- Nonlinear motion and mechanical mixing in as-grown GaAs nanowires
F. R. Braakman, D. Cadeddu, G. Tütüncüoglu, F. Matteini, D. Rüffer, A. Fontcuberta i Morral, and M. Poggio
Appl. Phys. Lett. 105, 173111 (2014). (pdf)

Nanometer-scale scanning SQUID microscopy
We develop and apply scanning SQUIDs for high-resolution magnetic and thermal imaging. We are one of the few groups world-wide to implement a technique – first developed by the Zeldov group at the Weizmann institute – in which a nanometer-scale SQUID is integrated onto a scanning probe. We have used these SQUID-on-tip devices to study the behavior of nanomagnets, superconducting vortices, and current flowing in superconducting qubit devices [4]. We now coordinate a European FET-Open project, ‘Focused ion beam fabrication of superconducting scanning probes (FIBsuperProbes)’, aimed at further developing on-tip superconducting probes. In this context, we have developed a new SQUID-on-lever probe, which combines the magnetic and thermal imaging provided by a an on-tip SQUID with the tip-sample distance control and topographic contrast of a cantilever used in atomic force microscopy. These devices are now being used in imaging experiments on nanomagnets and 2D materials.
References
- Imaging magnetic spiral phases, skyrmion clusters, and skyrmion displacements at the surface of bulk Cu2OSeO3
E. Marchiori, G. Romagnoli, L. Schneider, B. Gross, P. Sahafi, A. Jordan, R. Budakian, P. R. Baral, A. Magrez, J. S. White, and M. Poggio
arXiv:2407.05044. (pdf)
- Visualizing thickness-dependent magnetic textures in few-layer Cr2Ge2Te6
A. Vervelaki, K. Bagani, D. Jetter, M.-H. Doan, T. K. Chau, B. Gross, D. V. Christensen, P. Bøggild, and M. Poggio
Commun. Mater. 5, 40 (2024). (pdf) (pdf)
- Fabrication of Nb and MoGe SQUID-on-tip probes by magnetron sputtering
G. Romagnoli, E. Marchiori, K. Bagani, and M. Poggio
Appl. Phys. Lett. 122, 192603 (2023). (pdf) (pdf)
- Magnetic imaging of superconducting qubit devices with scanning SQUID-on-tip
E. Marchiori, L. Ceccarelli, N. Rossi, G. Romagnoli, J. Herrmann, J.-C. Besse, S. Krinner, A. Wallraff, and M. Poggio
Appl. Phys. Lett. 121, 052601 (2022). (pdf) (pdf)
- Magnetic, thermal, and topographic imaging with a nanometer-scale SQUID-on-lever scanning probe
M. Wyss, K. Bagani, D. Jetter, E. Marchiori, A. Vervelaki, B. Gross, J. Ridderbos, S. Gliga, C. Schönenberger, and M. Poggio
Phys. Rev. Appl. 17, 034002 (2022). (pdf)
- Nanoscale magnetic field imaging for 2D materials
E. Marchiori, L. Ceccarelli, N. Rossi, L. Lorenzelli, C. L. Degen, and M. Poggio
Nat. Rev. Phys. 4, 49 (2022). (pdf)
- Stray-field imaging of a chiral artificial spin ice during magnetization reversal
M. Wyss, S. Gliga, D. Vasyukov, L. Ceccarelli, G. Romagnoli, J. Cui, A. Kleibert, R. L. Stamps, and M. Poggio
ACS Nano 13, 13910 (2019). (pdf) (pdf)
- Imaging pinning and expulsion of individual superconducting vortices in amorphous MoSi thin films
L. Ceccarelli, D. Vasyukov, M. Wyss, G. Romagnoli, N. Rossi, L. Moser, and M. Poggio
Phys. Rev. B 100, 104504 (2019). (pdf) (pdf)
- Imaging stray magnetic field of individual ferromagnetic nanotubes
D. Vasyukov, L. Ceccarelli, M. Wyss, B. Gross, A. Schwarb, A. Mehlin, N. Rossi, G. Tütüncüoglu, F. Heimbach, R. R. Zamani, A. Kovács, A. Fontcuberta i Morral, D. Grundler, and M. Poggio
Nano Lett. 18, 964 (2018). (pdf) (pdf)
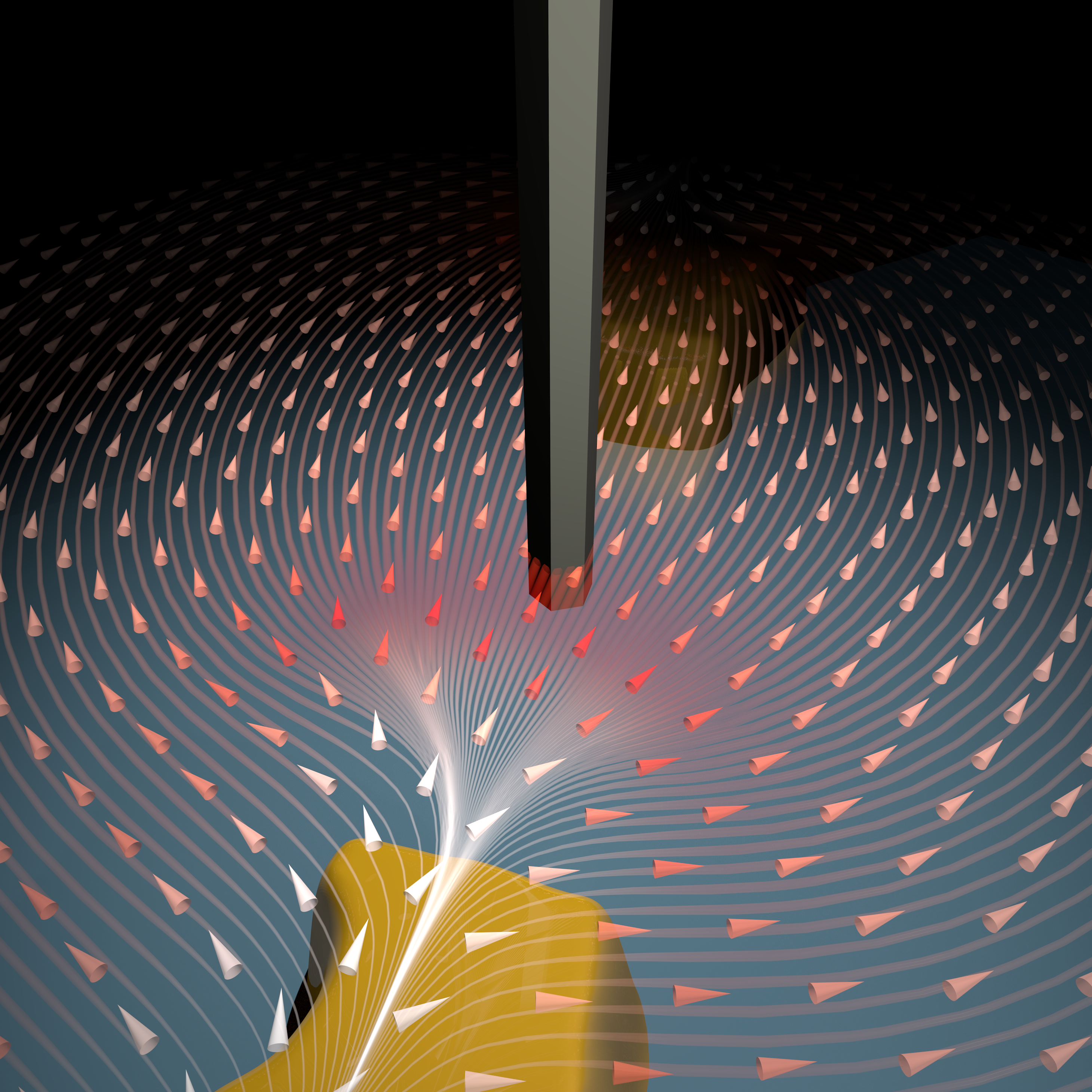
Nanometer-scale scanning probe microscopies
We develop both NW MFM and improving scanning SQUID probes by exploring new geometries and new superconducting materials, e.g. in an effort to increase the operating temperature of the SQUID probes above 77 K. As exemplified by our coordination of FIBsuperProbes, our vision is to enable a new era in scanning probe microscopy, in which nanometer-scale sensing devices can be directly patterned on-tip and used to reveal new types of contrast. One of the ways to realize this vision is via focused ion beam techniques to produce sensors with unprecedented size, functionality, and sensitivity directly on the tips of custom-designed cantilevers. We expect the resulting imaging techniques will significantly surpass state-of-the-art and help us to unravel poorly understood phenomena in physics, chemistry, and material science, which are impossible to address with today’s technology.
References
- Imaging magnetic spiral phases, skyrmion clusters, and skyrmion displacements at the surface of bulk Cu2OSeO3
E. Marchiori, G. Romagnoli, L. Schneider, B. Gross, P. Sahafi, A. Jordan, R. Budakian, P. R. Baral, A. Magrez, J. S. White, and M. Poggio
arXiv:2407.05044. (pdf)
- 2024 roadmap on magnetic microscopy techniques and their applications in materials science
D. V. Christensen, U. Staub, T. R. Devidas, B. Kalisky, K. C. Nowack, J.L. Webb, U.L. Andersen, A. Huck, D. A. Broadway, K. Wagner, P. Maletinsky, T. van der Sar, C. R. Du, A. Yacoby, D. Collomb, S. Bending, A. Oral, H. J. Hug, A.-O. Mandru, V. Neu, H. W. Schumacher, S. Sievers, H. Saito, A.A. Khajetoorians, N. Hauptmann, S. Baumann, A. Eichler, C. L. Degen, J. McCord, M. Vogel, M. Fiebig, P. Fischer, A. Hierro-Rodriguez, S. Finizio, S. S. Dhesi, C. Donnelly, Felix Büttner, O. Kfir, W. Hu, S. Zayko, S. Eisebitt, B. Pfau, R. Frömter, M. Kläui, F. S. Yasin, B. J. McMorran, S. Seki, X. Yu, A. Lubk, D. Wolf, N. Pryds, D. Makarov, and M. Poggio
J. Phys. Mater., DOI: 10.1088/2515-7639/ad31b5 (2024). (pdf)
- Mapping the phase-separated state in a 2D magnet
H. Mattiat, L. Schneider, P. Reiser, M. Poggio, P. Sahafi, A. Jordan, R. Budakian, D. V. Averyanov, I. S. Sokolov, A. N. Taldenkov, O. E. Parfenov, O. A. Kondratev, A. M. Tokmachev, and V. G. Storchak
Nanoscale 16, 5302 (2024). (pdf) (pdf)
- Scanning NV magnetometry of focused-electron-beam-deposited cobalt nanomagnets
L. Žaper, P. Rickhaus, M.Wyss, B. Gross, M. Poggio, and F. R. Braakman
ACS Appl. Nano Mater. 7, 3854 (2024). (pdf) (pdf)
- Nanoscale magnetism and magnetic phase transitions in atomically thin CrSBr
M. A. Tschudin, D. A. Broadway, P. Reiser, C. Schrader, E. J. Telford, B. Gross, J. Cox, A. E. E. Dubois, D. G. Chica, R. Rama-Eiroa, E. J. G. Santos, M. Poggio, M. E. Ziebel, C. R. Dean, X. Roy, and P. Maletinsky
arXiv:2312.09279. (pdf)
- Visualizing thickness-dependent magnetic textures in few-layer Cr2Ge2Te6
A. Vervelaki, K. Bagani, D. Jetter, M.-H. Doan, T. K. Chau, B. Gross, D. V. Christensen, P. Bøggild, and M. Poggio
Commun. Mater. 5, 40 (2024). (pdf) (pdf)
- Magnetic imaging of superconducting qubit devices with scanning SQUID-on-tip
E. Marchiori, L. Ceccarelli, N. Rossi, G. Romagnoli, J. Herrmann, J.-C. Besse, S. Krinner, A. Wallraff, and M. Poggio
Appl. Phys. Lett. 121, 052601 (2022). (pdf) (pdf)
- Magnetic, thermal, and topographic imaging with a nanometer-scale SQUID-on-lever scanning probe
M. Wyss, K. Bagani, D. Jetter, E. Marchiori, A. Vervelaki, B. Gross, J. Ridderbos, S. Gliga, C. Schönenberger, and M. Poggio
Phys. Rev. Appl. 17, 034002 (2022). (pdf)
- Nanoscale magnetic field imaging for 2D materials
E. Marchiori, L. Ceccarelli, N. Rossi, L. Lorenzelli, C. L. Degen, and M. Poggio
Nat. Rev. Phys. 4, 49 (2022). (pdf)
- Nanowire magnetic force sensors fabricated by focused-electron-beam-induced deposition
H. Mattiat, N. Rossi, B. Gross, J. Pablo-Navarro, C. Magén, R. Badea, J. Berezovsky, J. M. De Teresa, and M. Poggio
Phys. Rev. Appl. 13, 044043 (2020). (pdf)
- Stray-field imaging of a chiral artificial spin ice during magnetization reversal
M. Wyss, S. Gliga, D. Vasyukov, L. Ceccarelli, G. Romagnoli, J. Cui, A. Kleibert, R. L. Stamps, and M. Poggio
ACS Nano 13, 13910 (2019). (pdf) (pdf)
- Imaging pinning and expulsion of individual superconducting vortices in amorphous MoSi thin films
L. Ceccarelli, D. Vasyukov, M. Wyss, G. Romagnoli, N. Rossi, L. Moser, and M. Poggio
Phys. Rev. B 100, 104504 (2019). (pdf) (pdf)
- Imaging stray magnetic field of individual ferromagnetic nanotubes
D. Vasyukov, L. Ceccarelli, M. Wyss, B. Gross, A. Schwarb, A. Mehlin, N. Rossi, G. Tütüncüoglu, F. Heimbach, R. R. Zamani, A. Kovács, A. Fontcuberta i Morral, D. Grundler, and M. Poggio
Nano Lett. 18, 964 (2018). (pdf) (pdf)
- Electric field sensing with a scanning fiber-coupled quantum dot
D. Cadeddu, M. Munsch, N. Rossi, J. Claudon, J.-M. Gérard, R. J. Warburton, and M. Poggio
Phys. Rev. Appl. 8, 031002 (2017). (pdf) (pdf)
- Vectorial scanning force microscopy using a nanowire resonator
N. Rossi, F. R. Braakman, D. Cadeddu, D. Vasyukov, G. Tütüncüoglu, A. Fontcuberta i Morral, and M. Poggio
Nat. Nanotechnol. 12, 150 (2017). (pdf) (pdf)
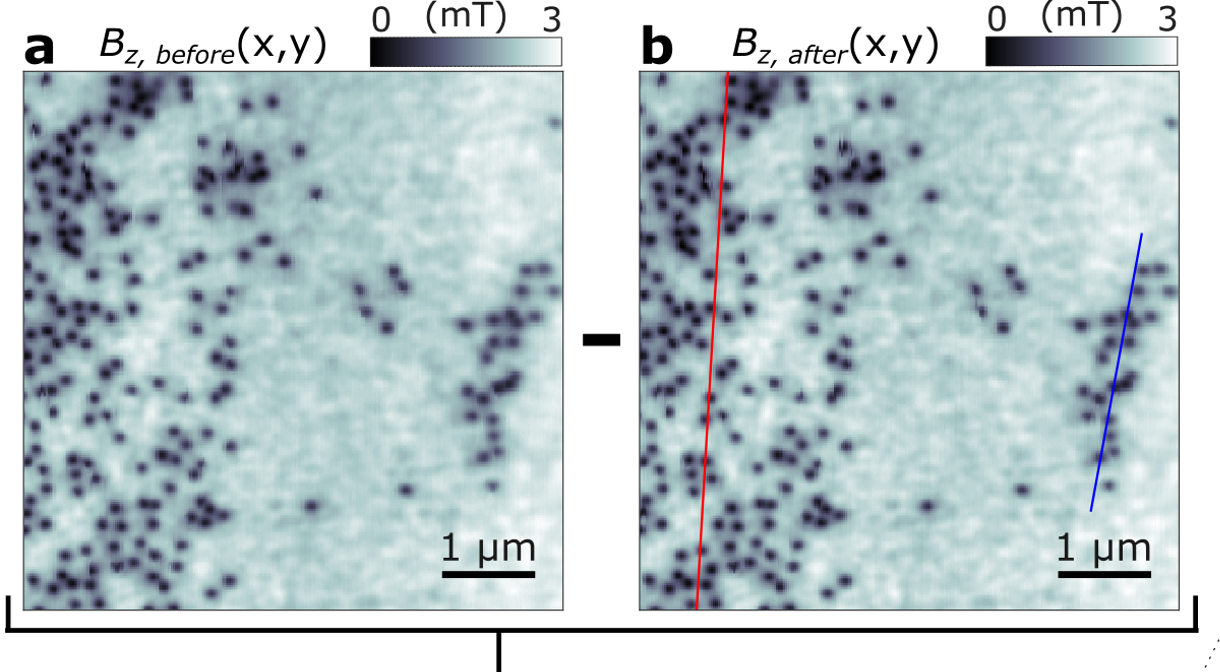
Magnetic skyrmions in bulk crystals
Their topologically protected spin-texture, which can be stable even at room temperature, their nanometer-scale size, and their manipulation via electric currents and fields make skyrmions a promising platform for information storage and processing. We have used sensitive torque magnetometry to measure the magnetic phase diagrams of nanostructured skyrmion hosts as well as small crystalline samples. We are now using scanning SQUID-on-tip probes to make real-space images of the magnetic field produced by skyrmions at the surface of bulk samples. This new application of scanning SQUID is starting to provide a microscopic spatial information on phase transitions, domains, domain walls, and the configuration of mixed magnetic phases in skyrmion hosting materials. It promises to bring new insight, which was – until now – experimentally inaccessible.
References
- Imaging magnetic spiral phases, skyrmion clusters, and skyrmion displacements at the surface of bulk Cu2OSeO3
E. Marchiori, G. Romagnoli, L. Schneider, B. Gross, P. Sahafi, A. Jordan, R. Budakian, P. R. Baral, A. Magrez, J. S. White, and M. Poggio
arXiv:2407.05044. (pdf)
- Stability of Néel-type skyrmion lattice against oblique magnetic fields in GaV4S8 and GaV4Se8
B. Gross, S. Philipp, K. Geirhos, A. Mehlin, S. Bordács, V. Tsurkan, A. Leonov, I. Kézsmárki, and M. Poggio
Phys. Rev. B 102, 104407 (2020). (pdf)
- Macroscopic manifestation of domain-wall magnetism and magnetoelectric effect in a Néel-type skyrmion host
K. Geirhos, B. Gross, B. G. Szigeti, A. Mehlin, S. Philipp, J. S. White, R. Cubitt, S. Widmann, S. Ghara, P. Lunkenheimer, V. Tsurkan, A. O. Leonov, S. Bordács, M. Poggio, and I. Kézsmárki
npj Quantum Mater. 5, 44 (2020). (pdf) (pdf)
- Stabilized skyrmion phase in MnSi nanowires detected by dynamic cantilever magnetometry
A. Mehlin, F. Xue (薛飞), D. Liang, H. Du, M. J. Stolt, S. Jin, M. Tian, and M. Poggio
Nano Lett. 15, 4839 (2015). (pdf) (pdf)
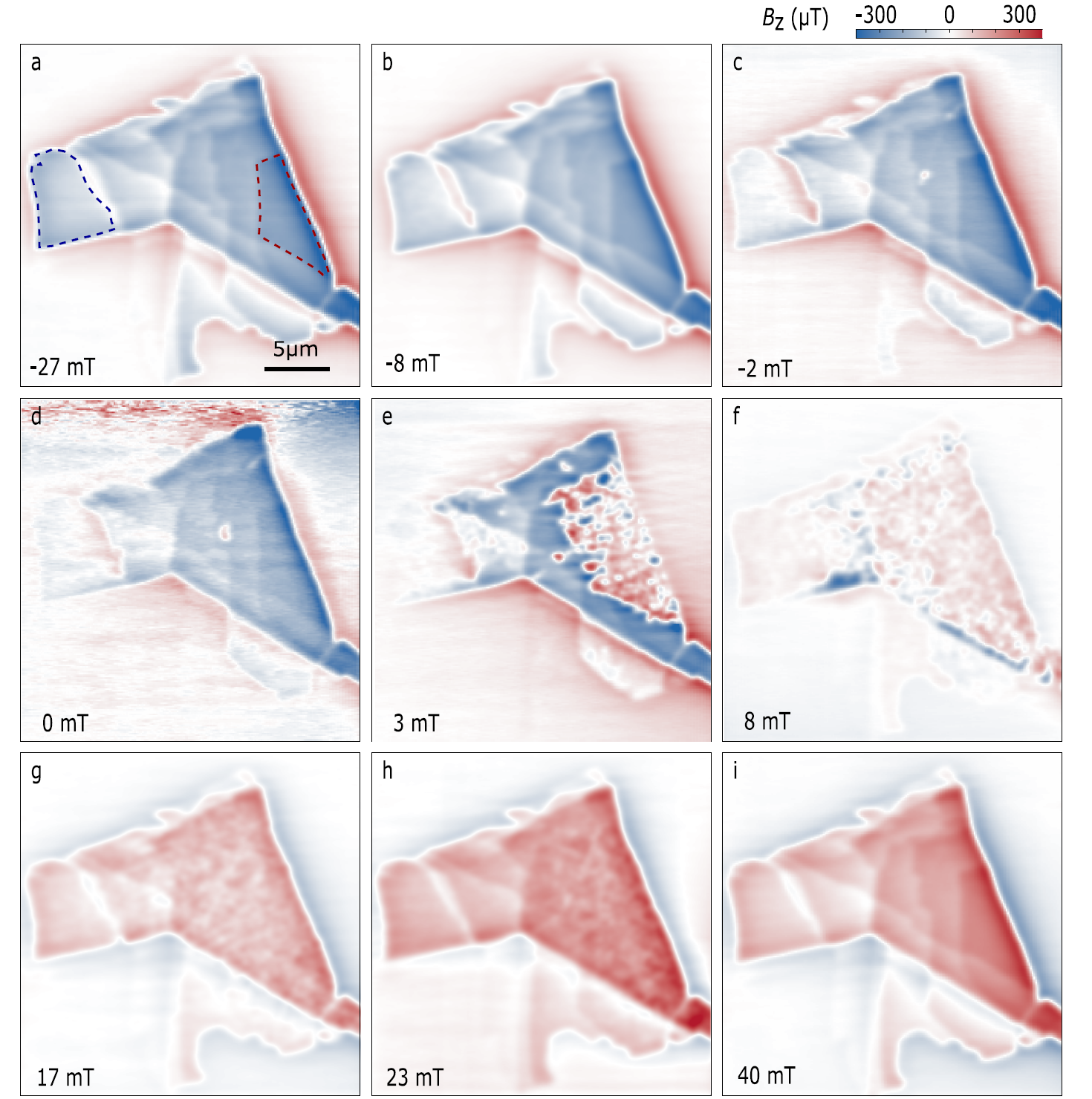
Real-space magnetic imaging of 2D magnets
2D magnets have emerged as a new frontier in magnetism, both in terms of fundamental questions as well as from a device engineering point of view. In order to take advantage of these new developments, we must understand the role of anisotropy, disorder, inhomogeneity, and characteristic length-scales in 2D magnets and their heterostructures. Such investigations require sensitive local probes and techniques for measuring magnetism in small volumes. We have started to apply our unique magnetometry and imaging tools to study static magnetization, phase transitions, magnetic reversal, and to understand how to control 2D magnetism.
References
- Mapping the phase-separated state in a 2D magnet
H. Mattiat, L. Schneider, P. Reiser, M. Poggio, P. Sahafi, A. Jordan, R. Budakian, D. V. Averyanov, I. S. Sokolov, A. N. Taldenkov, O. E. Parfenov, O. A. Kondratev, A. M. Tokmachev, and V. G. Storchak
Nanoscale 16, 5302 (2024). (pdf) (pdf)
- Nanoscale magnetism and magnetic phase transitions in atomically thin CrSBr
M. A. Tschudin, D. A. Broadway, P. Reiser, C. Schrader, E. J. Telford, B. Gross, J. Cox, A. E. E. Dubois, D. G. Chica, R. Rama-Eiroa, E. J. G. Santos, M. Poggio, M. E. Ziebel, C. R. Dean, X. Roy, and P. Maletinsky
arXiv:2312.09279. (pdf)
- Visualizing thickness-dependent magnetic textures in few-layer Cr2Ge2Te6
A. Vervelaki, K. Bagani, D. Jetter, M.-H. Doan, T. K. Chau, B. Gross, D. V. Christensen, P. Bøggild, and M. Poggio
Commun. Mater. 5, 40 (2024). (pdf) (pdf)
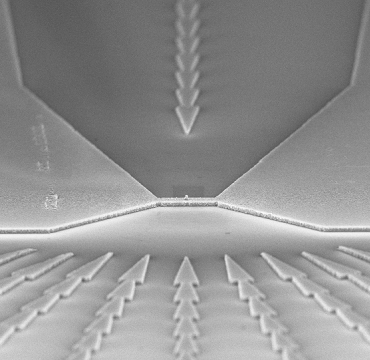
Magnetic resonance force microscopy
The proposal of magnetic resonance force microscopy (MRFM) and its subsequent realization combine the physics of magnetic resonance imaging (MRI) with the techniques of scanning probe microscopy. This pursuit is driven by the ultimate goal of imaging a single nuclear spin and the promise of a molecular structure microscope. The promise of this technique was demonstrated by a landmark experiment in 2004 in which an IBM group led by Dr. Dan Rugar measured the force of flipping a single isolated electron spin using a nanoscale cantilever. This achievement was not only among the first single-spin measurements, but also showed the versatility of nanoscale force sensors beyond conventional applications such as atomic force microscopy (AFM) and magnetic force microscopy (MFM). Progress continued with the report in 2009 of three-dimensional MRI of proton spins in a biological specimen with a spatial resolution under 5 nanometers.
We worked on improving MRFM detection sensitivity and on applying the method to nanometer-scale volumes of quadrupolar nuclear spins. Our demonstrated ability to detect nanometer-scale volumes of Ga and As nuclei using MRFM shows that — in principle — sub-surface, isotopically selective imaging of semiconductors nanostructures is possible. We also used MRFM to study the peculiar regime of nanometer-scale spin ensembles, in which statistical fluctuations in the nuclear polarization dominate the thermal polarization. More recently, our work on sensitive nanowire force sensors may eventually lead back to MRFM and to improvements in its sensitivity and resolution. As in any force microscopy, improvements in the force transducer are crucial for improving sensitivity and resolution.
References
- Roadmap on nanoscale magnetic resonance imaging
R. Budakian, A. Finkler, A. Eichler, M. Poggio, C. L. Degen, S. Tabatabaei, I. Lee, P. C. Hammel, E. S. Polzik, T. H. Taminiau, R. L. Walsworth, P. London, A. Bleszynski Jayich, A. Ajoy, A. Pillai, J. Wrachtrup, F. Jelezko, Y. Bae, A. J. Heinrich, C. R. Ast, P. Bertet, P. Cappellaro, C. Bonato, Y. Altmann, and E. Gauger
Nanotechnology, DOI: 10.1088/1361-6528/ad4b23 (2024). (pdf)
- Force-detected nuclear magnetic resonance
M. Poggio and B. E. Herzog
Micro and Nano Scale NMR: Technologies and Systems, J. Anders and J. Korvink, Eds., Wiley (2018), pp. 381-420. (pdf)
- Boundary between the thermal and statistical polarization regimes in a nuclear spin ensemble
B. E. Herzog, D. Cadeddu, F. Xue (薛飞), P. Peddibhotla, and M. Poggio
Appl. Phys. Lett. 105, 043112 (2014). (pdf)
- Harnessing nuclear spin polarization fluctuations in a semiconductor nanowire
P. Peddibhotla, F. Xue (薛飞), H. I. T. Hauge, S. Assali, E. P. A. M. Bakkers, and M. Poggio
Nat. Phys. 9, 631 (2013). (pdf) (pdf)
- Magnetic Resonance Force Microscopy
M. Poggio and C. L. Degen
Encyclopedia of Nanotechnology, B. Bhushan, Ed., Springer (2012), pp. 1256-1264. (pdf)
- Measurement of statistical nuclear spin polarization in a nanoscale GaAs sample
Fei Xue (薛飞), D. P. Weber, P. Peddibhotla, and M. Poggio
Phys. Rev. B 84, 205328 (2011). (pdf) (pdf)
- A geometry for optimizing nanoscale magnetic resonance force microscopy
Fei Xue (薛飞), P. Peddibhotla, M. Montinaro, D. P. Weber, and M. Poggio
Appl. Phys. Lett. 98, 163103 (2011). (pdf) (pdf)
- Force-detected nuclear magnetic resonance: recent advances and future challenges
M. Poggio and C. L. Degen
Nanotechnology 21, 342001 (2010). (pdf)